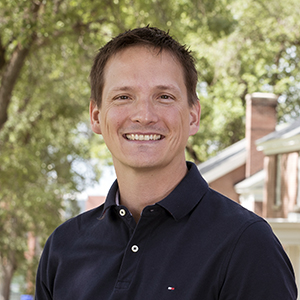
Dr. Gruenwald’s research predicting nanocrystal superlattices based on ligand interactions will receive NSF funding over the next five years to carry out his proposed plans to advance his field and educate the next generation of researchers.
“Investment in exemplary faculty members early in their careers is key to achieving the National Science Foundation’s mission to enhance the United States’ science and engineering ecosystem,” said NSF Director France Córdova. “Congratulations to this year’s CAREER awardees for their outstanding service as researchers and teacher-scholars. We look forward to your discoveries and innovations that will advance the frontiers of scientific understanding.”
This CAREER award supports theoretical and computational research and education on fundamental questions in nanoparticle self-assembly. Nanoparticles are tiny pieces of matter, consisting of only a few hundred to thousands of atoms, and can have extraordinary properties. When large numbers of nanoparticles are arranged in ordered patterns, called “superlattices”, they can act as highly efficient solar cells, can function as new types of sensors in medicine and technology, and can be used as filters to purify water and air. Because of their small size arranging nanoparticles into superlattices cannot be performed particle by particle. Instead, researchers utilize “self-assembly”, a process inspired by nature; self-assembly builds bio-molecular structures like biological cell. In self-assembly, nanoparticles are subjected to conditions that favor automatic formation of ordered patterns. Ideally, nanoparticles arrange themselves into the targeted superlattice due to mutually attractive forces. However, the forces between these tiny particles are not well understood and self-assembly often results in disordered structures or in patterns that are different from the desired one.
The most important forces between nanoparticles during self-assembly do not come from the particles themselves, but from so-called ligands, short chains of atoms that form a soft protective layer on the surface of nanoparticles. During self-assembly, ligands on nearby nanoparticles interact with each other in complicated ways that are difficult to probe in experiments. In this project, the PI and his team will develop new theoretical models and computer simulation methods that will increase understanding of ligand interactions. Computer simulations will be used to reveal how the length and number of ligands, as well as their interactions with the nanoparticles, determine what superlattice will form during self-assembly. To this end, new computational methods will be developed that allow the simulation of the self-assembly of large numbers nanoparticles and their ligands. The results of this research will provide a reference for future experiments and will help pave the way for more targeted self-assembly of nanoparticle superlattices; it will contribute to broad efforts to engineer improved devices based on nanoparticles.
Educational activities of this award focus on improving student success in an undergraduate Physical Chemistry class at the University of Utah. Research has shown that many students struggle with the abstract concepts and advanced mathematics of Physical Chemistry courses. The PI will address these challenges by developing a set of computer simulation exercises that are designed to illustrate key concepts of molecular fluctuations and help student build accurate mental models and reliable intuition for the behavior of atoms and molecules.